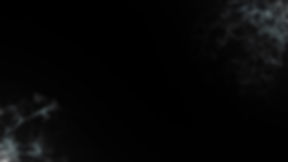
¿ What do we do ?
Understanding the properties and the changes of materials at the molecular level is the essence of chemistry. Theoretical chemistry explains the structural and physical properties of molecular systems comprising various materials and the chemical reactions governing the changes in those systems based on fundamental principles. Current trends in theoretical physical chemistry can be characterized by the efforts to provide much more direct and detailed information by mimicking the structures and motions of the real molecular systems. These advances are made possible by the recent developments in high speed and large capacity computer technologies. It is now possible to perform very efficient and accurate calculations when applying quantum, classical, and statistical mechanics to molecular systems with increasing number of particles. This new field of chemistry, where the properties of materials are examined computationally without carrying out real experiments, is called Computational Chemistry. Computational chemistry is one of the most rapidly growing fields with increasing applications in related areas such as physics, astronomy, biology, medical/pharmaceutical sciences and material sciences. While the traditional molecular modeling methods are usually concerned with the predictions of static properties, the main focus of our research is to study dynamical processes involved in the chemical changes leading to specific functions of molecular systems. The purpose of the research is, first, to develop theories, mathematical frameworks and computational methods for describing quantum dynamics of complex molecular systems. The dynamical processes accompanying chemical reactions under various conditions are treated by efficient theoretical methods according to the complexities of the dynamics. The second area of research concerns with the applications of computer simulations such as Molecular Dynamics and Monte Carlo simulations to chemical reactions in condensed matter, self-assembly at surfaces/interfaces, functional mechanism of biological enzymes, and understanding of protein folding.
​
1. Quantum Control of Molecular Dynamics by Laser Pulses
We have used theories and computational methods of quantum dynamics in order to examine salient features of various chemical reaction dynamics. Efficient computational methods such as multiple-time-scale quantum wavepacket propagation, combined quantum/classical mechanical methods, and semi-classical methods have been developed to extend the range of systems to be studied. We have also developed new theoretical concepts and computational methods for studying reaction dynamics where the nonadiabatic processes due to the coupling of several electronic states play important roles. These theoretical approaches were applied to charge transfer reactions, which play important roles in many physical/chemical/biological phenomena. The effects of environments on charge transfer processes were examined in the study of photodissociation and recombination of molecular ions in clusters or condensed matter. One-electron model was proposed to provide theoretical framework to understand the interplay between the charge transfer dynamics and the nonadiabatic phenomena due to the strong interactions among electronic states. Electron and proton transfer are closely related with photosynthesis and respiration, which are involved in the energy transfer phenomena in biological systems. We have studied a model proton-coupled-electron-transfer reaction in order to provide theoretical background in understanding such phenomena. Active control of chemical reactions, which may be termed as Modern alchemy, based on the fundamental knowledge of the reaction dynamics at the molecular level will open up unlimited possibilities for future application.
​
​
​
​
​
​
​
​
​
​
​
​
​
​
​
​
​
​
​
​
​
2. Molecular Dynamics
Self-assembly is ubiquitous in biological molecules and other materials in nature. Understanding self-assembly process is not only essential for solving the mysteries of life, but also important in providing efficient means of synthesizing new materials with designed functionality. Langmuir monolayers of amphiphiles or macromolecules at interfaces have drawn much attention due to their potential use in applications such as electro-optical materials and chemical/biological sensors as well as their importance as model systems for biomembranes. Our group has been actively involved in the theoretical and computational studies of Langmuir monolayers and other related systems such as Self-Assmbled Monolayers. The main purpose of the research is to perform systematic studies on the interplay between the molecular architectures and macroscopic properties, the characteristics of phase transitions, and the effect of substrate in monolayer systems. Recently, for the first time, we have examined the detailed dynamical processes involved during the formation of Langmuir monolayers by implementing new methods of Molecular Dynamics simulations. Systematic researches on monolayers are expected to provide advanced knowledge and control over nanoscale architectures, which are essential for designing brand new functional materials for wide spectrum of applications.
The outstanding potential of Computational Chemistry is most apparent in the field of biological sciences. The relation between the structure and function of biological molecules can be examined rather accurately by the current technologies in molecular modeling and computer simulations. Ultimately, the analysis of the vast genetic information and the prediction of the structures and specific functions of proteins will be tackled by efficient computational methods. We have been pursuing active researches in the applications of theories and computational tools of quantum and molecular dynamics to the bio-macromolecules. We have developed new ways of examining function-related structural changes and interactions from the MD simulations. These theoretical methods have been applied to study the mechanism of enzymes such as Lipase and to elucidate the characteristic structural changes in proteins due to mutations. In the wake of the completion of Genome project, one of the most important problems in Computational Chemistry will be to understand and predict the Protein folding process. New theoretical and computational approaches are needed to tackle such challenging problems. Applications of efficient sampling schemes such as multicanonical ensemble method are being investigated. Building very powerful parallel machines such as Linux clusters are expected to contribute significantly for the computational studies of function-related structural changes in proteins.
3. Electronic Structure
Electronic structure calculation is nowadays a powerful tool for chemists. It is considered to be 'accurate' and extensively used in many fields including nano- and bio-science. Under frozen-nuclei approximation, it mainly deals time-independent properties like molecular structure and energy. With some perturbation, dynamic properties like transition probability can also be obtained.
We focus on the study of nanostructures and reactions of biological molecules. Techniques like QM/MM method and density-functional theory, rather than full quantum-mechanical method, are used for such large molecular systems.
We are also interested in organic and/or inorganic reactions, which requires high-level quantum chemical calculations. Multi-reference calculation method using GAMESS code is currently adopted.
4. Bioinformatics
miRNA functional annotation
​
MicroRNAs (miRNAs) constitute an important class of regulators that are involved in various cellular and disease processes. However, the functional significance of each miRNA is mostly unknown due to the difficulty in identifying target genes and the lack of genome-wide expression data combining miRNAs, mRNAs and proteins. We introduce a novel database, miRGator, that integrates the gene expression data, target prediction, functional analysis and genome annotation. MiRNA function is inferred from the list of target genes predicted by miRanda, PicTar, and TargetScanS programs. Statistical enrichment test of target genes in each term is performed for gene ontology, pathway, and disease annotations. Associated terms may provide valuable insights for the function of each miRNA. For the expression analysis, miRGator integrates public expression data of miRNA with those of mRNA and protein. Expression correlation between miRNA and target mRNA/proteins is evaluated and their expression patterns can be readily compared. Our web implementation supports diverse query types including miRNA name, gene symbol, gene ontology, pathway, and disease terms. Interfaces for exploring common targets or regulatory miRNAs and for profiling compendium expression data have been developed as well. Currently, miRGator, available at http://genome.ewha.ac.kr/miRGator/, supports the human and mouse genomes.
Overview
miRNA biogenesis
​
MicroRNAs (miRNAs), a family of small noncoding RNAs of ~22 nucleotides in length, constitute an important class of regulators that are involved in diverse cellular processes such as developmental control, apoptosis, cell differentiation and proliferation. They are also implicated in various disease processes thus emerging as potential targets of intervention. The biogenesis of miRNA takes place in a stepwise manner. A long primary transcript (pri-miRNA) is processed by the nuclear RNase type III enzyme Drosha to release 60~70 nucleotides-long intermediates. It is often the case that several pre-miRNAs originate from a single polycistronic pri-miRNA. Consistently, miRNA genes are often found as clusters in narrow genomic regions. The intermediates, known as pre-miRNAs, have a hairpin structure whose base-pairing is not perfectly complementary with many mismatches and bulges. Pre-miRNAs are further processed to the mature mi-RNA by another RNase enzyme known as Dicer.
